Every year, 40,000 Canadians go into cardiac arrest and most of the time, there is no one around to call for help. As the seconds tick by and the brain is deprived of oxygen, a person’s chances of survival decline dramatically: fewer than three per cent of unwitnessed cases survive.
But what if we had a device that could detect cardiac arrest and immediately call 911?
This is the vision of Jacob Hutton and Dr. Mahsa Khalili, trainees at the Centre for Health Evaluation & Outcomes Sciences (CHÉOS) housed at St. Paul’s Hospital.
“Cardiac arrest is technically a death state and needs to be reversed by treatment, but nearly half of cardiac arrests don’t receive any treatment at all, often because they’re not detected,” says Hutton, a paramedic with BC Emergency Health Services and a PhD student supervised by CHÉOS’ Dr. Brian Grunau. “This is something we could move the needle on with some technological innovation.”
Through their affiliation with the BC Resuscitation Research Collaborative (BC RESURECT), co-led by CHÉOS Scientists Drs. Jim Christenson, Brian Grunau, and Christopher Fordyce, along with BCEHS Chief Medical Officer Dr. Mike Christian, Hutton and Dr. Khalili are working to develop, validate, and implement wearable sensors to detect out-of-hospital cardiac arrest (OHCA).
“Knowing the impact of timely detection of out-of-hospital cardiac arrest and how many lives we can save is a great motivation,” says Dr. Khalili, a postdoctoral fellow in the Faculty of Medicine at UBC. “I also have a personal connection with this work: I lost my cousin to cardiac arrest.”
To illustrate the potential impact of detecting OHCA in BC, the group presented a modelling project at the Canadian Arrhythmia Network Symposium late last year and at the recent UBC Faculty of Medicine Research Day.
“We looked at all unwitnessed cases in BC in 2019 and 2020 and asked ‘What would be the incremental increase in survivorship if 10 per cent of them had a sensor, all the way up to 100 per cent?’,” explains Hutton. “We modelled the effect of recognition without any bystander intervention; the isolated effect of having someone witness the arrest and then call 911.”
The group found there would be as many as 900 additional survivors in the province over the two-year period had all unwitnessed cases been equipped with a cardiac-arrest device. Other estimates suggest that the widespread use of biosensors would increase the number of cardiac arrest survivors nationally from 1,800 to 6,000 per year. The projection is a strong example of their potential. In the real world, the group doesn’t expect everyone to wear a sensor.
“This type of modeling work is usually the first stage when you’re trying to look at implementing technologies,” says Hutton. “We’re asking: ‘What could we achieve if we actually were able to roll this out?’”
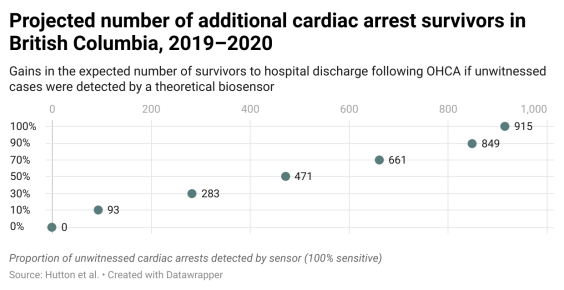
How biosensors can act as a bystander during cardiac arrest
In their modelling study, the researchers also looked at how varying levels of sensitivity affect performance.
“Sensitivity is what people most commonly associate with accuracy. It’s the ability of the sensor to detect a cardiac arrest when it occurs,” says Hutton.
While ensuring that these devices don’t miss a cardiac arrest and a chance to save a life, the rate of false positives (known as specificity) is what could make or break the project.
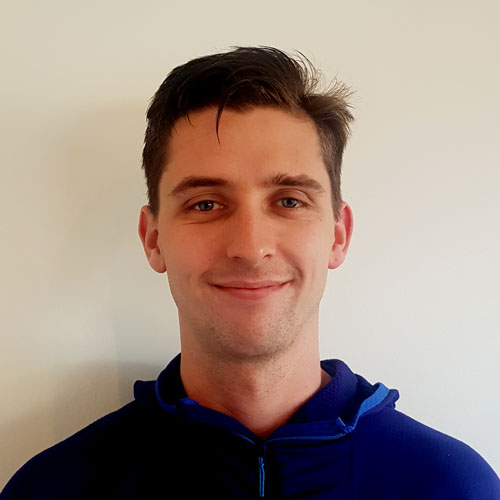
“When you’re wearing these devices 20 hours at a time, one false positive per day per person could result in so much extra demand to the already overburdened EMS system that it would kill any chance of implementing these devices at a large scale,” explains Hutton.
Accordingly, balancing the sensitivity and specificity is a primary focus of the researchers, which is where Dr. Khalili and the engineering team come in.
“The technology we are focusing on is similar to what’s already used in a smartwatch like the Apple Watch or Fitbit,” says Dr. Khalili, a member of the Implantable Biosensing Laboratory and the Human Motion Biomechanics Laboratories at UBC. She is co-supervised by Dr. Grunau, along with Drs. Babak Shadgan and Calvin Kuo of the UBC School of Biomedical Engineering.
While devices like the Apple Watch are being used to detect cardiac arrhythmia, Dr. Khalili says there are currently none on the market that can detect cardiac arrest. There are devices in development that can be used for cardiac arrest detection, but they mostly rely on a single sensor or one type of sensor.
“Instead of relying on one source of information to detect a cardiac arrest, we are focusing on combining multiple sensors to improve the performance or the prediction of cardiac arrest,” she said.
This multimodal approach is something that is used in other devices, including the Apple Watch’s arrythmia tool, but they are unable to continuously monitor the wearer.
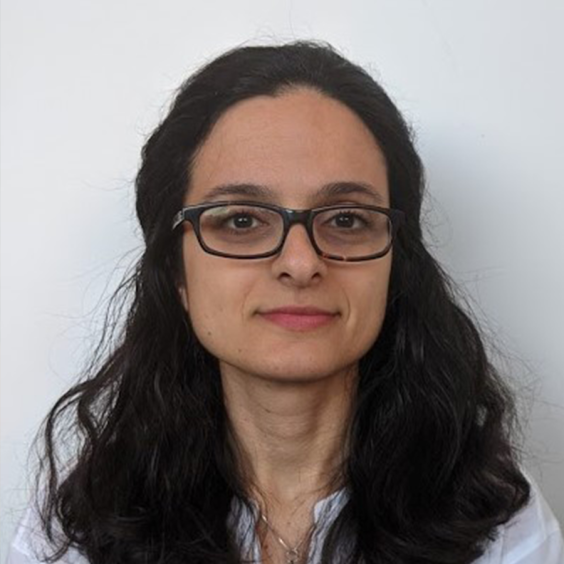
“The Apple Watch has a motion sensor, an optical sensor, and an ECG to measure cardiac electrical activity, but while the motion and optical sensors collect continuously, the ECG requires the user to initiate the measurement and remain stationary for 30 seconds,” explains Dr. Khalili. “This is not feasible during cardiac arrest, so an ideal device needs to have all these sensor types, all in place, with continuous monitoring.”
This multimodal approach helps to ensure that a cardiac arrest is not missed, but it still doesn’t help address the risk of false positives, one of the biggest issues in the field, according to Dr. Khalili. She’s working to address the specificity issue by improving the algorithms integrated with these technologies to provide more accurate detection of OHCA.
“Our short-term goal is to achieve 98-per-cent specificity with our wearables,” says Dr. Khalili. In other words, two per cent of the time an alert is triggered, the sensors will falsely indicate a cardiac arrest. “But this is still not optimal performance for real-world implementation.”
Device design and theory can only go so far, and tuning the sensors and algorithm requires testing under some unusual circumstances.
How to test real-world effectiveness
One of the major hurdles in this work is that the team can’t feasibly perform a large-scale, randomized, controlled trial to test these sensors in the real world.
“In the traditional clinical trial model, we would have to randomly assign 200,000 biosensors to potentially get a statistical signal that we could detect,” explains Hutton.
Instead, they are focusing on small studies in high-risk cardiac arrest patients, as well as studies that simulate cardiac arrest, both in animal models and humans.
“We are planning to test our devices in high-risk human participants; this is the closest that we can get to validate both the sensors and the algorithms,” says Dr. Khalili.
Eventually, the devices could be tested in the real world by having a registry of people wearing them, which would be tied to an alerting system to trigger a response from emergency services.
The way these devices could be integrated into emergency systems is a key component of this work.
“Sensors are integrated into devices, and then devices into systems. Eventually, we would like to develop a device-agnostic system; one that can take data from multiple devices, assimilate it, make a decision, and contact 9-1-1,” says Hutton.
They are also interested in exploring the integration of the sensors into crowdsourced bystander CPR apps, like the Pulse Point or Good Sam apps, where people in the community could get an alert on their phone and then respond to a cardiac arrest.
The team is also developing their own app alongside their device for users to provide their exact location, address, and details to improve emergency services response times, a crucial component to improve chances of survival.
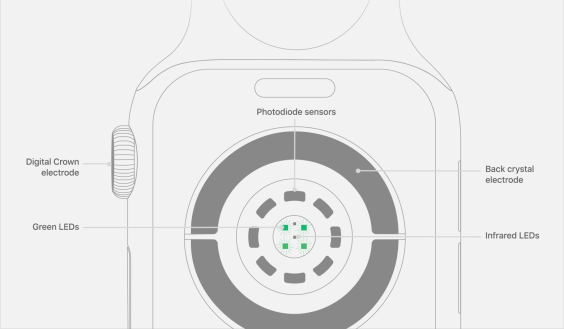
The integration of these devices into emergency systems is ultimately where the team envisions their main focus to be. To this end, they are currently pursuing industry and commercial partnerships. “It’s very unlikely from a funding and development perspective that we will create our own biosensor, market it, and get it out into the public at a level that would be able to compete with what’s currently out there,” says Hutton.
With a focus on integration across the full chain, from sensor to device to system, Jake says that this is where they can leverage their expertise.
“This is a huge dark area for most people who are doing this work in industry,” he adds. “Understanding the feasibility constraints and interface with 911 is where we provide differentiated value from a lot of these people who are focused on using these devices as a diagnostic tool in isolated clinical settings.”
This article was adapted from the original CHÉOS story by Sean Sinden, here.